After the Fukushima incident in Japan in March of 2011, where the nuclear power plant was hit by a tsunami, scientists used numerical models of ocean currents to predict the possible speed and direction of radioactive materials that had been released.
Yongqi Gao, a scientist at both the Nansen Center and the Bjerknes Center, is one such researcher who has worked with simulations of ocean currents and the flow of radioactive isotopes from Fukushima into the Pacific Ocean.
Radioactive material has also been released from the Sellafield nuclear power station in England. From this site, Gao followed the isotope Cs-137 in order to understand its flow pattern along particular ocean currents. From the Sellafield plant on the north-western coast of England, these isotopes typically take four years until the reach the Norwegian coast.
Useful waste for researchers
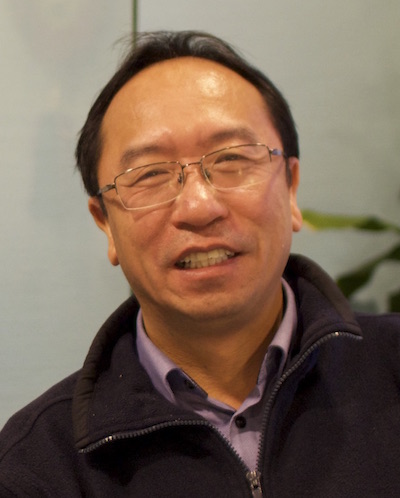
Yongqi Gao is involved in the ORGANIC research project which started in 2015. He is working on including biogeochemical tracers in an ocean circulation model to understand the formation and movement of deep ocean currents.
Gao has previously simulated CFC’s (chlorofluorocarbons) used in commercial products such as Freon, the greenhouse gas SF6 used in the electrical industry, and the radioactive isotopes Cs137 and Sr90 as tracers in an Ocean General Circulation Model (OGCM). Cs-137 and Sr-90 are radioactive isotopes released into the environment from nuclear power plants and nuclear weapons testing.
“Since they are passive tracers, they can be used to assess the simulated ocean circulation. For CFCs, the scientific community also uses them to estimate the oceanic uptake of anthropogenic CO2,” says Gao.
Deep ocean currents
The world’s ocean currents flow across vast distances, acting as global transport systems. These currents interact with regional climate patterns along the way. Warmer currents are driven along the ocean surface by wind, while deep water currents are driven by differences in temperature and salinity in what is known as thermohaline circulation (thermo- referring to heat and -haline to salt).
In the northern Atlantic, cold, dense water sinks from the surface to the deeper ocean and eventually forms the North Atlantic Deep Water (NADW). Quantifying the NADW formation and its pathways is tricky since the NADW lies almost two thousand meters below the surface.
Models to predict future events
Results from tracer simulations are usually compared with observed data. These kinds of studies help researchers to evaluate and adjust their models, which in turn leads better ocean current models.
Similarly, a better understanding of the movement of deep ocean currents will help researchers to improve predictions of long-term climate effects.
Gao’s colleague in the ORGANIC project, Jerry Tjiputra, created the animation below, which shows a simulation of the tracer CFC-11’s penetration and movement in the Atlantic over the last few decades since its initial release into the atmosphere.
[https://vimeo.com/145392498]
CFC11_Animation from The Bjerknes Centre on Vimeo.
The animation’s colour scale indicates the concentration of CFC-11 at different depths across the Atlantic.
The spread of CFC-11 is shown on a grid of the Atlantic Ocean in the bottom left. Along these points, the penetration depth is simulated with the results on the panel on the right, which also compares the results using two different model resolutions.
Comparing the two simulations, you will see a high concentration of CFC-11 in both is found at a depth of 1 000m and between 30deg-40deg north, roughly between the latitudes of Madrid and New Orleans.
What impacts changes in ocean circulation?
These simulations are run using the oceanic component of the Norwegian Earth System Model (NorESM). NorESM is a coupled model for the entire global physical climate system, including the carbon cycle, and is the product of collaboration between various Norwegian institutes.
The model is complex and a part of Gao’s work is to identify uncertainties in the NorESM by using tracers in the model. Together with colleagues Yanchun He and Mats Bentsen, he forms a multi-disciplinary research team studying the sensitivity and rate of thermohaline ocean circulation.
They are currently focusing on simulating tracer movement within the NADW, which includes using CFC’s to quantify the amount of diffusion that occurs under different conditions.
“The changes in the horizontal and vertical diffusion/mixing can lead to changes in the water density, and by that to changes in ocean circulation.”
“The North Atlantic Deep Water is the lower-limb of the Atlantic Meridional Ocean Circulation (AMOC),“ and “the change in AMOC has an impact on climate,” explains Gao.
Reference:
He Yanchun, Gao Yongqi, Wang Huijun, Johannessen Ola, Yu Lei (2012): Transport of Nuclear Leakage from Fukushima Nuclear Power Plant in the North Pacific. Acta Oceanologica Sinica, 34(4),12-20 (in Chinese with abstract in English)